Ground potential differences can wreak havoc on precise oscilloscope measurements, especially with single-ended scopes- where you will always have an offset error in your readings.
To illustrate the problem, we will start from a very simple measurement and work our way into more complex, realistic scenarios, intentionally making things erroneous by pushing what would happen.
The scenario used includes a PC providing 5 volts from a USB hub. We will measure that 5v using a single-ended scope (like a common desk scope). For simplicity and illustration, we will use an ideal 5v source so all distortions we see on the simulated scope traces will be from external factors.
Envision plugging the Tek scope into a 120v wall outlet, and our PC into a different wall outlet upstream from the Tek, but on the same 120v branch circuit. For simplicity, we will use 120v RMS DC instead of 120v RMS AC as our wall outlet power. We don’t want to model rectifiers in the Tek or PC; but the effect on the scope traces using DC instead of AC will be similar; similar enough that it will make clear what is going on.
We will pick currents and wire sizes that are realistic, but intentionally in a direction to best illustrate measurement distortions. For our simulations, we will say the PC draws a nominal current of 3As and the oscilloscope draws a nominal current of 1A. These are modeled as current sources in the simulation.
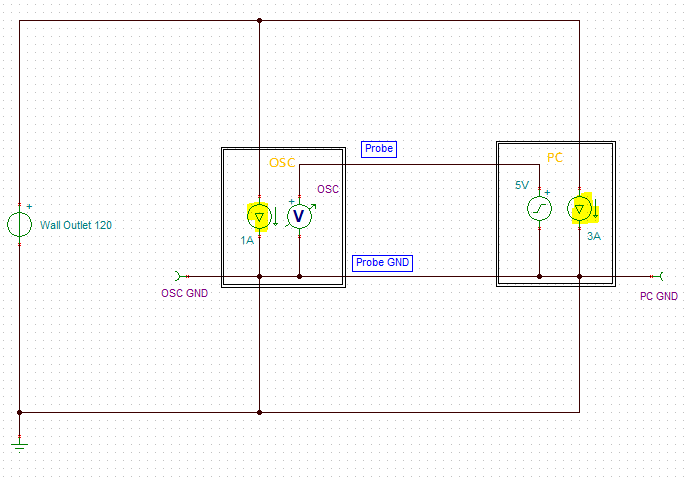
Our simulated oscilloscope is at the OSC (probe) in the schematics. And as expected, the measured value on our oscilloscope is a nice steady 5v.

But this really isn’t reality. In reality there is some resistance in our probe wires. Typically probe wires are small; let’s assume 24 AWG and a probe length of 3 feet.
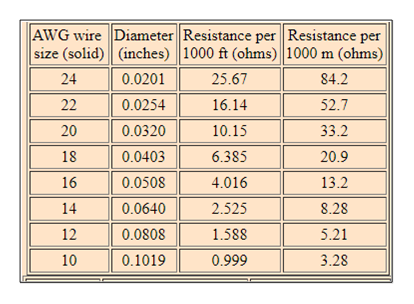
Given standard wire gauge resistances, we will have a resistance of about 77mΩs. (3*25.67/1000 ~ 77mΩ).
Let’s redraw our circuit including the 77mΩ probe wire resistance.
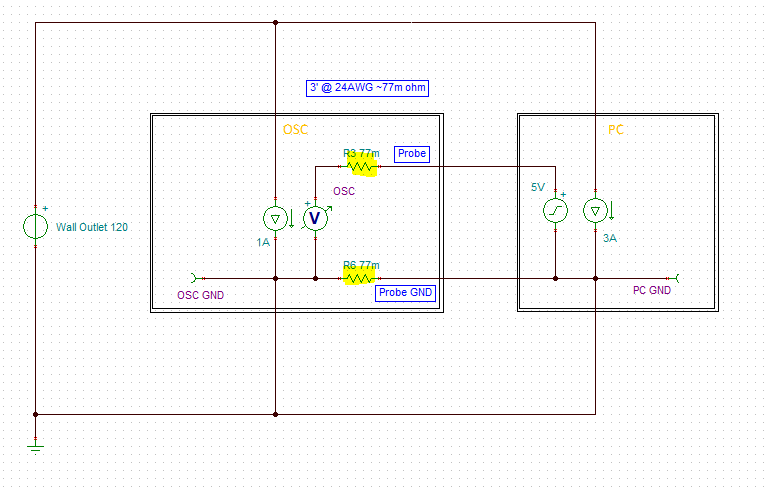
And again we get a nice steady 5v measurement on our oscilloscope.

But this isn’t reality. In reality there is some resistance in our power cord that plugs the PC and the oscilloscope into the wall. We will assume the power cords are 18 AWG and are 6 feet long for both. Using our chart, the power cords will present about 38mΩs of resistance (6*6.385/1000 = ~38mΩ).
Let’s redraw our circuit including the 38mΩ power cord resistance.
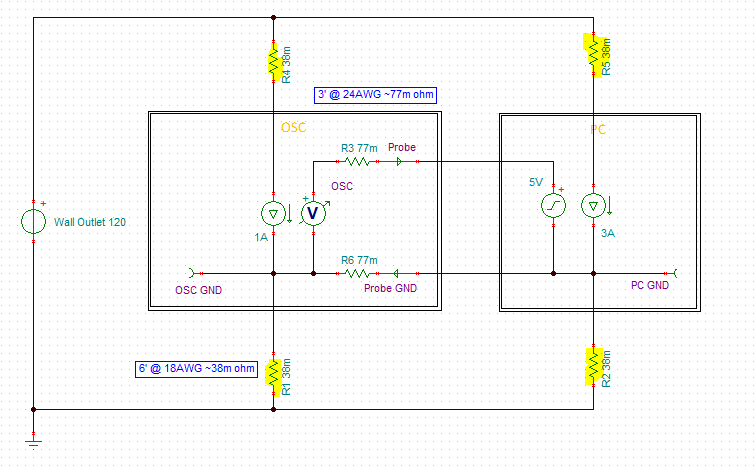
But now we see a 40mV offset error in our oscilloscope measurement.
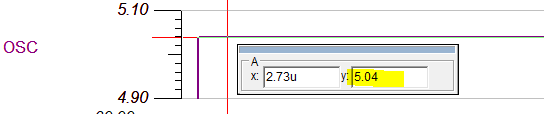
If we look at the Probe ground current, we see that there is 500mA (1/2amp) of current going through our little tiny 24 AWG probe ground wire. There is almost no current going through the positive probe wire.
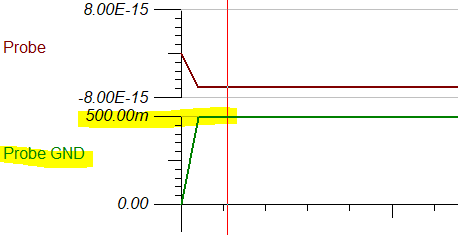
What is happening, the oscilloscope and the PC have different internal ground potentials because of the IR drop in their respective power cords. We can see there is a 38.25mV different (our 0.5a * 77mΩ = ~38mV)
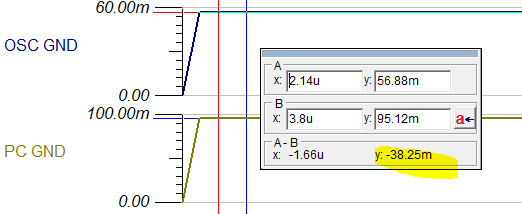
But again, this isn’t reality. In reality, there is some resistance in the wall branch circuit between the PC and the oscilloscope. Now a 15 amp wall branch circuit will typically use 14 AWG wire. Let’s assume that our PC is upwind of our oscilloscope by 10 feet between 2 wall outlets. Given our chart, that would yield a wall resistance of about 25mΩ (10*2.525/1000 = ~25mΩ).S
To continue, let’s update our schematic to include the 25mΩ wall resistance between our instruments.
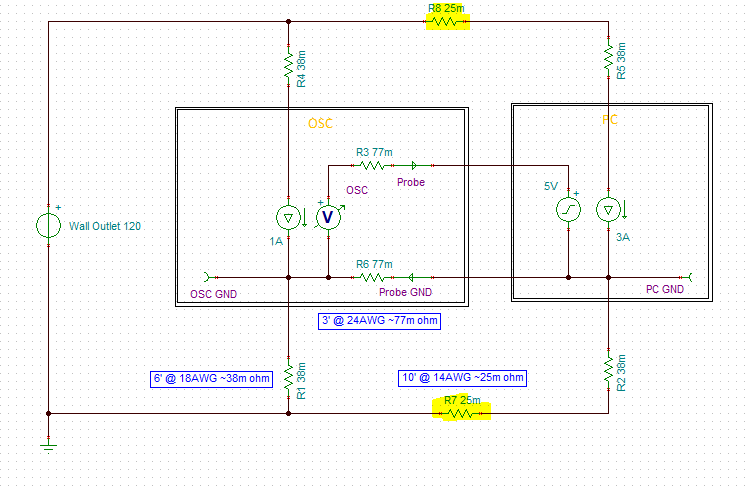
We now measure 5.07v on the oscilloscope, a 70mV offset error. Our 24 AWG Probe ground wire is passing 848mA, and the difference between the PC and oscilloscope internal grounds is now 65mV.
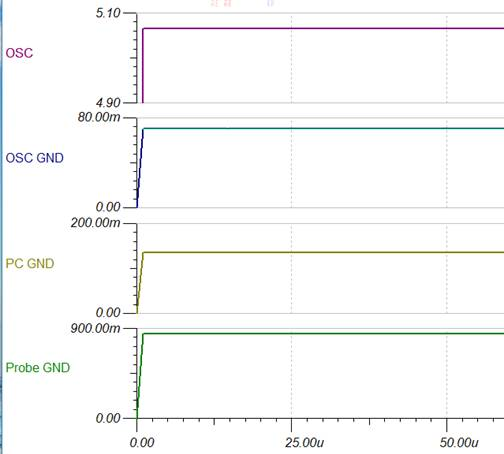
But this still really isn’t reality. In reality, there is probably something else plugged into our wall branch circuit besides just the PC and oscilloscope. Our wall circuit can provide 15 amps from the branch circuit breaker. Our PC takes 3A, or oscilloscope takes 1A, let’s add something big upstream on the branch circuit, say a 7A appliance.
Let’s add that to our circuit.
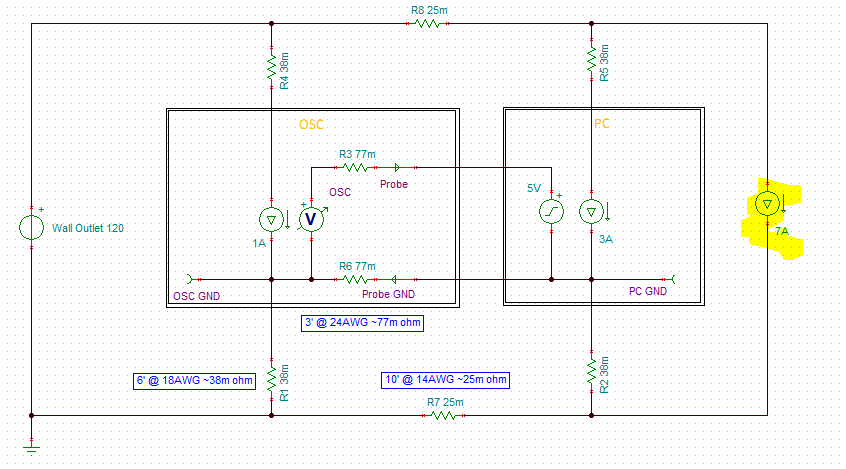
Now we measure 5.14mV on our oscilloscope, an offset error of 140mV! Our 24 AWG probe ground wire is passing 1.83 amps! That probe ground wire might be getting warm!
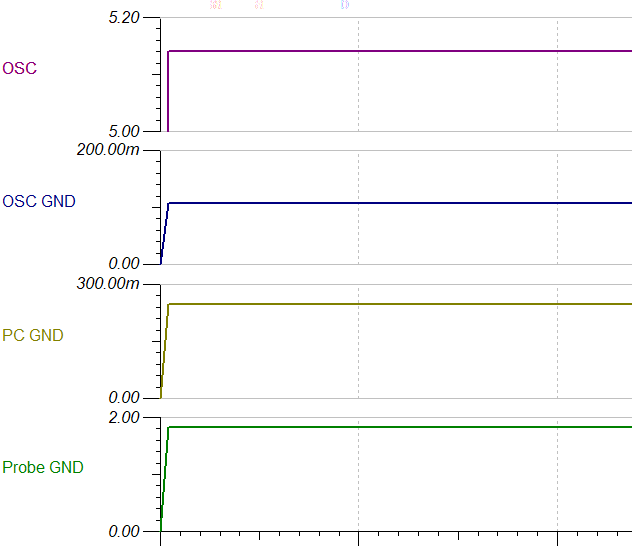
But this really isn’t reality. In reality there is probably some switching noise going on in the PC and oscilloscope. Let’s simulate that with a 500mA 100kHz square wave on the PC, and 250mA 500kHz triangle wave on the oscilloscope.
Let’s add that to our circuit.
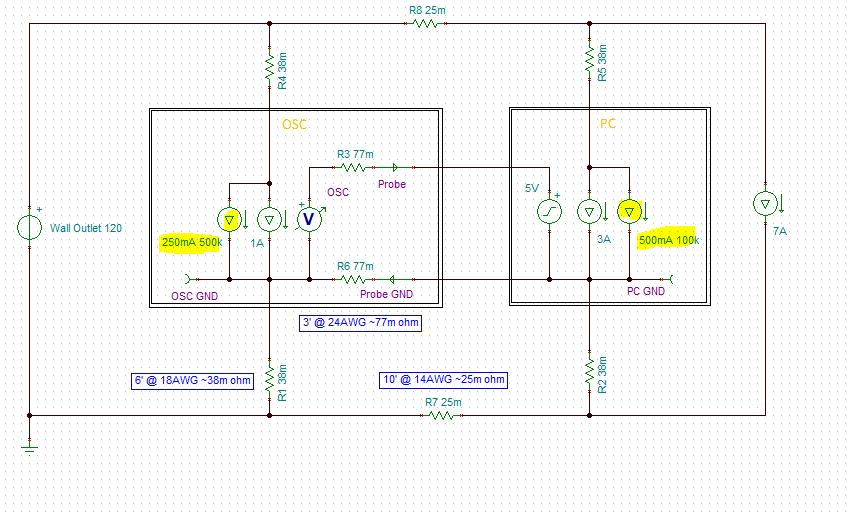
We see a 34.6mV ripple on our oscilloscope measurement!
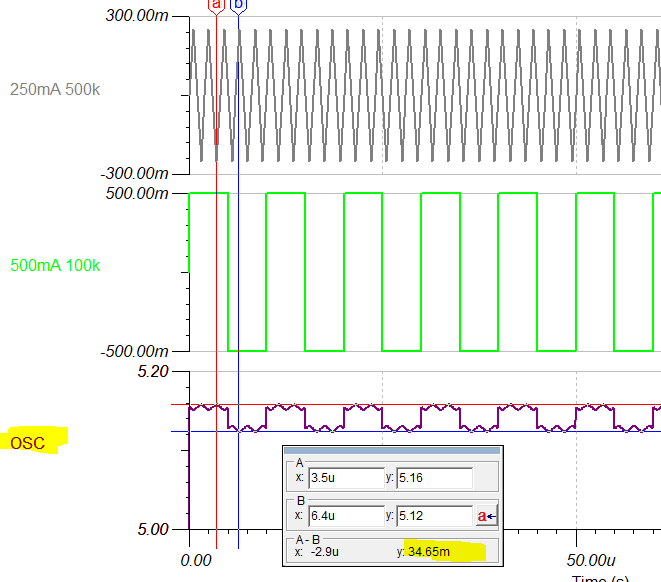
But let’s not stop just yet! Let’s add upstream on our wall branch circuit a noisy appliance. Now typically it would probably be at 60Hz, but that is going to be hard to show, so we are going to put a 3A 200kHz sine wave appliance on the circuit. We picked 200kHz so we can easily see the effects on our simulated oscilloscope trace. In reality, it probably would be a much lower frequency appliance, but the effect would still be there.
For fun, let’s add that to our wall branch circuit.
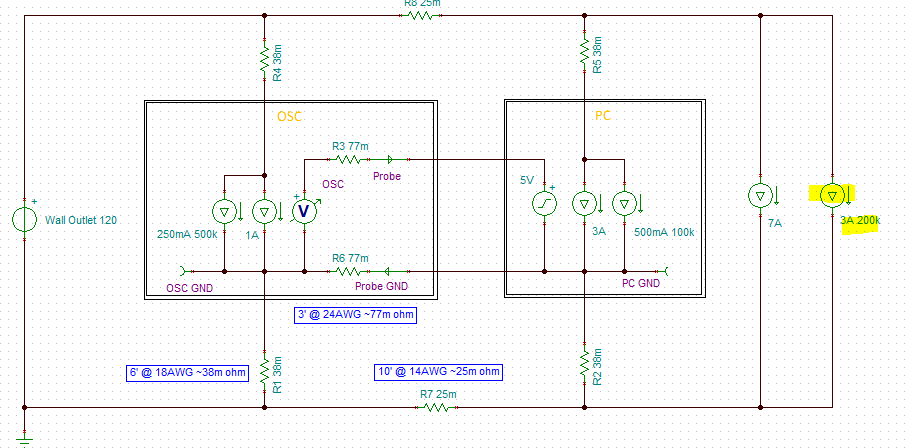
Now we have nearly 100mV of ripple measured on the oscilloscope and a really ugly looking waveform!
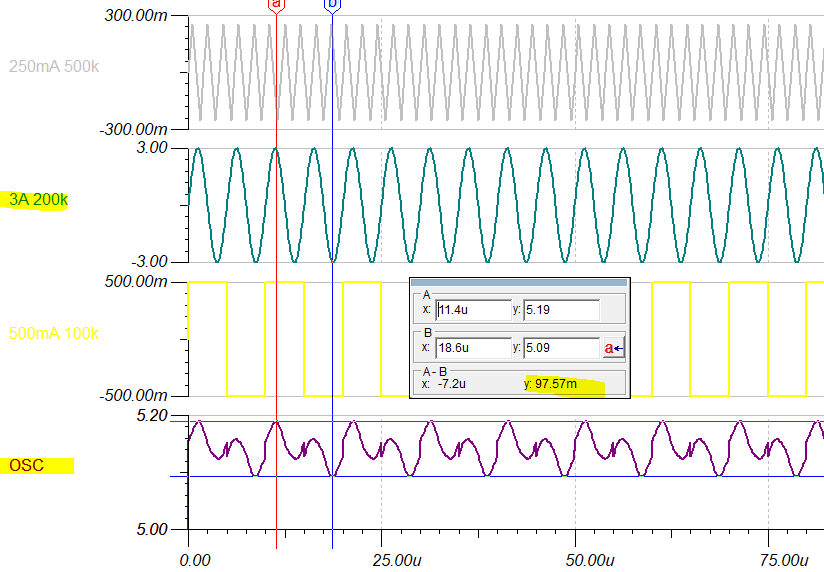
So here we are, using our nice expensive Tektronix’s oscilloscope, attempting to measure a nice steady 5v source from our PC; and what do we see? We see a messy signal offset by 140mV!
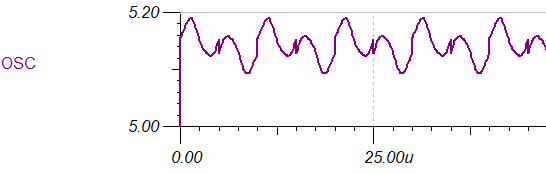
Our nice 5v output doesn’t look so nice anymore, but it really isn’t real, it is a scope measurement distortion due to differentiating ground references.
Now let’s take a look at what we have on my desk; a real world condition. Let’s measure my PC USB ground against our Tektronix’s ground (ground to ground) with my Agilent DMM (which has an isolated ground). We see a 95mV of ground differential between the PC and the Tek. This is due to the IR drop in the power cords and in the wiring in the wall, the branch circuit.
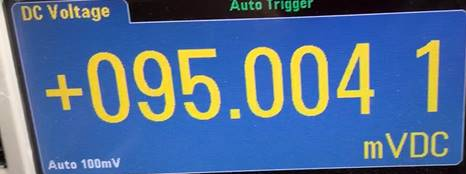
But, beware; even though this shows 95mV of difference, as soon as I hook up the probe ground wire, the grounds will equalize via the probe’s ground wire and the difference will be greatly reduced. But, there will be an IR drop across the probe ground wire and that is what will show up as an offset error on the scope trace. For example, when we hook up the probe ground to my PC, my offset error drops to 5.5mV; and in reality, we typically see anywhere from 5-15mV of offset error on my Tek.
Using a differential probe (ground to ground), we can see hundreds of mVs of noise (probably switching noise). This will all show up as additional noise on any scope trace.
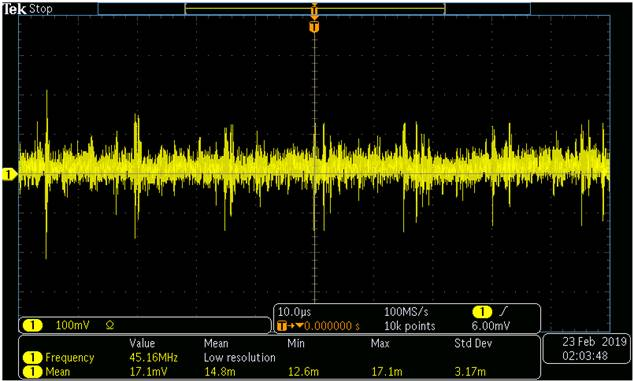
So how do we fix this? How do we see what is really there?
- Ideally, if we could get the scope and the unit under test to have the same ground potential, and without switching noise, our single-ended scope would work just fine.
- One thing we can do is couple the grounds of the scope and the unit under test with a very heavy gauge wire; reducing, but not illuminating, the ground differences.
- Use a scope with an isolated ground.
- This will allow the scope to float to the same ground potential as the unit under test.
- There will still be some capacitive coupling that can allow interference to affect the signal measurement, but usually this is minimal.
- The OpenScope and OpenLogger can have isolated grounds by powering them off of a battery and using WiFi to talk to them. This completely isolates the scope from any external interference.
- This will allow the scope to float to the same ground potential as the unit under test.
- Use a differential probe
- This allows one probe to trace the unit under test’s ground, while the other probe measures the signal relative to that ground.
- But differential probes are expensive. If I need one, I have to borrow it.
- Use averaging on the scope to remove noise, and know what the nominal offset error is.
- This is what I do most of the time.
- Sometimes, you just don’t know what is real, and what is interference. Judgment and discussions with others are the best that can be done.
This blog post is courtesy of Keith Vogel.